The Forward Scattering Spectrometer Probe (FSSP)
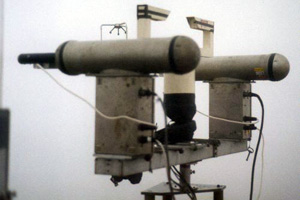
A forward scattering spectrometer probe (FSSP) is an optical particle counter, suitable for counting and sizing particles in the size range of 0.5 - 47 micron diameter. It essentially consists of a laser, various optics, a photo-detector module, and electronics to process the data. The UMIST FSSP is a PMS FSSP model 100 with the DMT SPP-100 rev-1 electronics upgrade, and is configured for ground based use.
Air is drawn through the inlet by an aspiration fan mounted behind the sample tube, at a rate of 25m/s. As droplets pass through the laser beam, the laser light is scattered. This scattered light falls on a photodiode (the direct beam falls on a dump spot) and the electronics register the presence of a particle. The intensity of the scattered light is determined by the size of the particle, its refractive index, and collection angle used, according to Mie scattering theory, the theoretical Mie curve is show in figure 2, along with the approximation used by the FSSP set up for 20 channels on range 0.
The electronics measure both the height of the pulse produced by the particle, and its duration. The maximum pulse height is latched, and this value digitised. Particle size is determined from the pulse height by comparing the digitised pulse height with a predetermined set of heights forming the upper limits of a number of size channels. The electronics then register one count in the size channel into which the pulse falls. After the designated time period the total number of particles detected in each size channel is transferred to the computer. The DMT electronics upgrade makes it possible for the user to define the number of channels, and the thresholds for each, for the refractive index of the particles to be measured. A combination of Mie Scattering Theory and glass bead calibrations are used to define the channel thresholds.
The FSSP laser beam does not have a uniform cross section being less intense towards the edges than in the centre, hence particles passing through the edges will be undersized. These particles are rejected, the portion of the cross section for which particles are accepted is defined as the active beam fraction. Particles passing through the beam at different points along its path have different collection angles, and are sized differently. A small area along the length of the beam, at the centre of the sample tube is defined as the depth of field. The active beam fraction and the depth of field define the probe sample area. Only particles passing through the sample area are counted and sized. The size of the sample area is measured when calibrating the probe.
Particles passing through the edges of the beam will spend less time in the beam, hence these can be distinguished from those passing through the centre. A running mean is maintained of the length of time taken for each particle to pass through the beam, particles which pass through the beam quicker than this average are rejected. Rejected particles are not sized, but a count is maintained of the number of particles rejected by this method.
Whether a particle has passed through the depth of field is determined by splitting the scattered light between two photo-detectors and comparing their output. One of the detectors is masked to block light being scattered from the central portion of the beam. If the signal from the masked detector exceeds that of the other detector, the particle is depth of field rejected. Again rejected particles are not sized, but a count is maintained of the number of particles rejected by this method.
For droplet concentrations and cloud liquid water content to be calculated the sample volume frequency of the probe must be known. This is the product of the sample area of the beam and the air speed through the inlet. Though the airspeed is nominally set at 25m/s by the aspiration fan, variations in wind speed cause variations in inlet airspeed. Wind speed is measured continuously using a sonic anemometer, and a correction applied to the air speed. The wind ramming correction applied is that derived empirically at UMIST in wind tunnel tests, and is given in the equations below.
A further complication with FSSP measurements is that it is a single particle instrument, i.e. when a particle is in the beam, and while the probe electronics are still busy processing data, it cannot simultaneously measure another one. Errors due to these effects are known as dead time, or coincidence errors. Electronic dead time is eliminated with this version of the probe by the use of FIFO buffering. Since the average transit time of droplets through the beam is recorded, the standard activity correction for coincidence errors is applied. This is given below.
In addition to the obvious effect of coincidence errors on measured droplet concentrations, in severe cases there may also be an effect on the size of the droplets. For there to be any effect on sizing, the second droplet must arrive before the peak intensity due to the first particle has been attained. The probability of this happening is low except in cases of very high droplet number. Under these circumstances it is very difficult to obtain good droplet size spectra, and liquid water content measurements from FSSP measurements. However, the droplet numbers measured are still reliable.