Optical Particle Counters
Introduction
Particle measurements using optical instruments are based on the fact that when a particle passes through a beam of light, some of the light is scattered. Detection of this scattered light is the basis of all such instruments. Particle number can be determined simply by counting the pulses of scattered light reaching the detector. However, it is possible to obtain much more information using optical scattering techniques than just number. The intensity of scattered light is related to the size of the scattered particle and this relationship can be used to make measurements of particle size. Further the spatial scattering pattern is dependant on particle shape, so this is another parameter which can be measured with optical instruments.
Size dependant scattering by spherical particles
The relationship between particle size and scattered intensity at any given scattering angle is complex and is fully defined for spherical particles of any size by Mie Theory. Depending on the size of the particle relative to the wavelength of the incident light there are simplifications which may be used to relate particle size to scattered intensity. The size parameter is defined by:
Where α is the size parameter, Dp is the diameter of the scattering particle and λ is the wavelength of the light.
There are three distinct scattering regimes:
α<<λ Rayleigh Scattering
Where I is scattered intensity, I0 is incident intensity, θ is the scattering angle, R is the distance to the particle, λ is the wavelength of the light, n is the refractive index of the particle and d is the diameter of the particle. For any given detector system and refractive index scattered intensity varies with d6 in this regime.
α~λ Mie Scattering
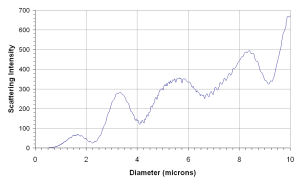
The relationship between particle diameter and scattered intensity in the Mie Scattering regime is much more complicated than in either of the other regimes. Figure 1 gives an example of the relationship between diameter and scattering intensity for water droplets.
α>>λ Simplified Geometric Scattering
I = I0K(n,θ)d2
where I is scattered intensity, I0 is incident intensity, K is a function of refractive index (n) and scattering angle (θ), and d is the particle diameter.
A full analysis of geometric scattering is also able to closely reproduce the results of Mie Scattering for smaller particles with a significant saving in computation (see Glantschnig and Chen 1981).
Most optical particle counters operate with visible or near infra-red wavelengths (typically 500-1100nm), putting most of the aerosol size range in the Mie Scattering regime. For some instruments measuring larger cloud and precipitation drops the simplified geometric relationship is applicable and for instruments measuring the smallest aerosol, Rayleigh scattering is applicable.
Practical Optical Particle Counters
As can be seen from the Mie curve shown, there is not a simple relationship between particle size and scattered intensity. However, with good optical design (choice of solid collection angle etc) the ambiguities in the Mie curve can be reduced, and useful size information obtained. In practise, theoretical instrument response curves are not used for sizing of particles, rather a series of calibration particles of known size are used to determine the response of the instrument over its full size range. A curve is fitted to these measurements to allow sizing of particles which fall between the calibration points.
In order to detect scattering pulses from single aerosol particles with the types of detector readily available an intense light source must be used. For this reason most optical particle counters use laser light to illuminate the particles. In order to measure smaller and smaller particles light intensity and detector sensitivity must be increased as at small sizes the relationship between particle size and scattered intensity starts to follow the Rayleigh law. To obtain the light intensity needed to measure particles below about 100nm with a laser system small enough to use in a portable instrument, the intra-cavity technique is used.
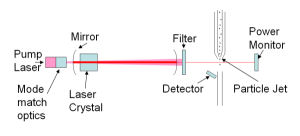
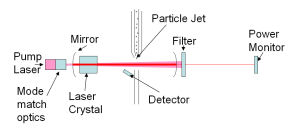
Schematic of a high power diode pumped solid state laser system of the type used in some optical particle counters illustrating the difference between standard and intracavity systems.
Most types of laser operate within a laser cavity, consisting of high reflectivity mirrors at each end of the cavity with the laser medium in between. Energy is applied to excite the laser medium, which then gives off light. This light is retained within the cavity stimulating the emission of more light and an intense coherent beam is formed along the axis of the cavity. A standard laser beam is formed by allowing a small quantity (typically <1%) of this light to escape through one of the mirrors. In general, any obstruction of the intra-cavity beam will prevent the laser from operating. However, the attenuation of the beam caused by aerosol particles passing through it is small enough not to affect the operation of the laser. Thus particles can be subjected to radiation >100 times more intense using an intra-cavity arrangement than would be possible using a standard arrangement of the same power. This results in a similar increase in signal strength. This technique is illustrated in figure 2, which shows a solid state intracavity system typical of the UHSAS and SP2 instruments. Many standard OPC's actually use a simpler laser than the one illustrated in the figure, typically using the output of a diode laser to illuminate the particles directly.
The intensity of scattered light from a 20µm particle is approximately 10 orders of magnitude larger than that from a 50nm particle. This is far beyond the dynamic range of a single detector or linear amplifier, placing practical limitations on the size range of particles which can be measured with a single detector. Thus in some cases it is a choice of whether to measure large or small particles, but in other instruments multiple detectors or amplification systems are used to extend the size range which can be measured.
In the above discussion of the relationship between size and scattering intensity, spherical particles have been assumed. However the way a particle scatters light is also strongly dependant on its shape. While some instruments are designed to minimise the effect of particle shape by integrating over a wide range of scattering angles, others use the differences in scattering to differentiate between particle shape, often employing multiple detectors to acheive this, though this is currently not possible for particles in the sub micron size range.
Optical particle Counters available at the Centre for Atmospheric Science
The Centre for Atmospheric Science makes regular use of several instruments whose operation depends on the principles outlined above. These are briefly described in the following paragraphs.
Ultra High Sensitivity Aerosol Spectrometer (UHSAS)
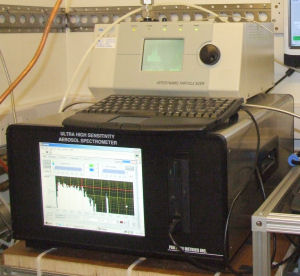
This instrument manufactured by Droplet Measurement Technologies is able size and count particles in the 50-800nm size range, and produces a very high resolution size distribution having 100 size bins. The instrument is able to operate at 10Hz, so is ideal for making particle flux measurements. The system is controlled and logged by a PC integrated into the instrument, which is also able to control other instrumentation in a stand alone flux system. This instrument uses a high power intra-cavity scattering system, with two separate detectors of different types, each with two different gains to obtain a wide size range. This instrument represents a major advance from its predecessor the PMS ASASP-X in terms of minimum particle size and size resolution.
PCASP (Passive Cavity Aerosol Spectrometer Probe)
The PCASP uses a passive cavity (so called because it contains no gain medium) external to the laser cavity, bounded with an oscillating mirror to increase laser intensity experienced by particles passing through the cavity by about 30%. 3 gain stages operating off a single detector are used to obtain a particle size range of 0.1-3microns with up to 40 size channels and a 20Hz data rate. This probe is usually operated as an airborne instrument mounted in a standard under wing canister.
Aerosol Spectrometer Probe
A prototype instrument manufactured with a size range of 0.3-3um, 40 size bins and a 10Hz time response. This instrument is small and lightweight making it ideal for aircraft use.
GRIMM Dust Monitor
Model 1.105, 1.108 and 1.109 Dust Monitors, manufactured by GRIMM Aerosol Technik GmbH. [Link]. These instruments have a size range of 0.3-20um, 15 size bins, and a 6s time response (1s response is possible with the 1.108, but with a limited size range). These instruments are small and self contained, being battery powered and having on board logging to a memory card. These instruments have been widely used in urban pollution projects mounted in a variety of locations around the city, and are also suitable for personal exposure measurements.
References
Werner J. Glantschnig and Sow-Hsin Chen. Light scattering from water droplets in the geometrical optics approximation. Applied Optics, Vol. 20, Issue 14, pp. 2499-2509. 1981.